Posphorus – the essence of life
Introduction
Phosphorus is an essential element for all carbon-based lifeforms on earth and is mostly present in the form of phosphates in the earth crust (Holleman et al. 1985). Phosphates are serving different functions in living organisms: They act as a backbone in the structure of DNA and RNA, they are necessary for the formation of bones in vertebrates and they play an important role in the energy- storage and supply of cells (Nigg and Herzog 2007). These phosphates are chemically bound together with other minerals, known as phosphate rock, with apatite being the most common mineral containing phosphates (Filippelli 2008). Phosphate rock is mainly sourced from deposits of minerals with a high concentration of phosphates. Through refining and chemical processing phosphoric acid can be produced from phosphate rock which then can be further processed (Mew et al. 2018). Phosphates are nowadays mainly used for fertilizer production in order to increase crop yields in agriculture and to a lesser extent as a component in detergents due to its chemical properties (Belboom et al. 2015; European Commission 2015). Due to chemical weathering, phosphates are present in marine, freshwater and terrestrial ecosystems and are commonly the limiting macronutrient for growth (Elser J.J. et al. 2007). Due to its specific properties as a chemical element and its importance to living organism phosphorus cannot be substituted by any other material (Edgerton 2009; Ye et al. 2017).
Due to above mentioned reasons phosphorus deserved its status as a critical raw material necessary for the development of a sustainable society. Therefore, it is important to assess the future availability, allocation and demand in order to explore future pathways to a sustainable supply. In 2014 the European commission did not yet include phosphorus in its list of critical materials, however the importance of phosphorus and its status as a critical raw material was later in 2017 recognized (European Commission 2020).
Present situation
Supply
Primary
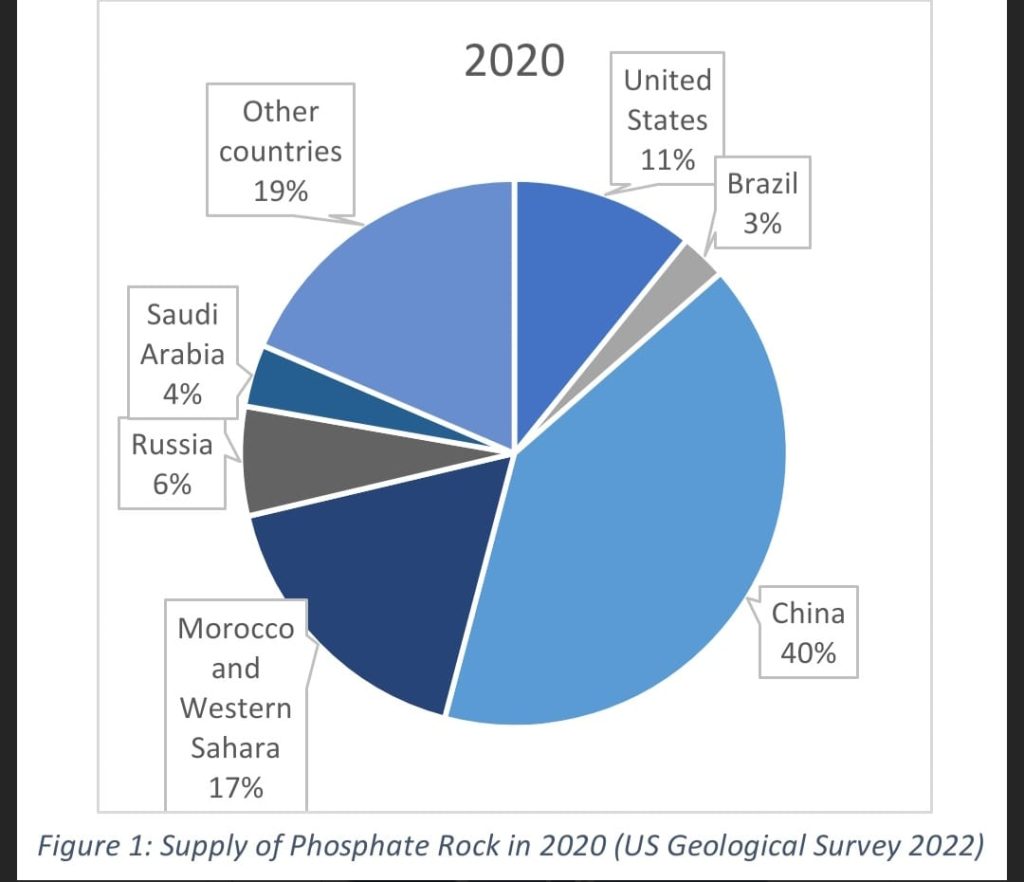
The primary supply nowadays is almost exclusively met by exploiting current known phosphate rock reserves of geological origin. These deposits were created by marine sedimentary sequences or through magmatic activity (Pufahl and Groat 2017). Historically, the phosphate-rich guano from bird and bat excrements was also an important source of phosphates but these deposits were depleted by the late 1800s and are not of any importance nowadays (Stewart et al. 2005; van Enk et al. 2011). Presently, countries supplying major quantities of phosphate rock are China, Morocco and Western Sahara, the Unites States, Russia, Saudi Arabia and Brazil. As depicted in Figure 1 in 2020 these countries provided 81 % of the global phosphate supply. Besides these, many more countries, especially in Asia, Africa and South America, produce notable quantities of phosphate rock (US Geological Survey 2022).
Secondary
Secondary supplies of phosphates basically comprise any waste streams from the technosphere containing phosphates such as sludge from wastewater treatment plants, greywater, manure from animal feedstocks, animal waste and agricultural residues (Cordell et al. 2011). The most important secondary supply sources utilized nowadays are of all agricultural nature including sludge from wastewater treatment plants (Shiu et al. 2017; Ye et al. 2017), livestock manure (Haase et al. 2017) and dry waste (Pearce and Chertow 2017). However, as stated by Elser and Bennett 2011 only 10 % of human waste from wastewater treatment plants is returned to agriculture to date. Without precaution, the direct utilization of phosphate-containing waste streams on agricultural fields can lead to negative effects such as an accumulation of heavy metals or of antibiotics or other pharmaceutical degradation products (Lamastra et al. 2018; Michael et al. 2013).
Reserves
A reserve is a known deposit of phosphate rock which is economically extractable. These reserves of phosphate rock are dynamic as the viability of extraction depends on the price of a material, the costs to extract it and the accessibility of a deposit (US Geological Survey 2022).
Current global primary reserves are estimated to be around 71 000 000 megatons from which 70 % are located in Morocco and Western Sahara. Other notable reserves are located in China, Egypt, Algeria, Australia, Brazil, Finland, Jordan, Saudi Arabia and South Africa. Large unclaimed reserves have also been discovered on continental shelves and on seamounts in the Atlantic and the Pacific Ocean (US Geological Survey 2022).
Demand
The demand for phosphate rock is mainly due to fertilizer production which accounts for 80 – 90 % globally (Lauriente 2003; Smil V. 2000). Other important applications are its use as animal feed and detergent (European Commission 2020). Recently, the EU is implementing policies which aim to reduce the amount of phosphor in detergents (European Commission 2015).
There is also a lot room for improvement concerning the use of phosphorus in fertilizers: According to The United Nations Food and Agriculture Organization only 15 – 30% of phosphates in fertilizers applied on fields are taken up by crops (FAO 2006). Furthermore, as stated by Cordell et al. 2009 about 55 % of the mined phosphor is lost “between farm and fork” due to inefficiencies in processing, transportation and storage.
As stated by Scholz et al. 2013 the demand of fertilizer globally differs: In the 1980s the fertilizer consumption in the industrialized world has stagnated and after the 1990s it even decreased to some extent. An increase in phosphorus use efficiency, increased quantities of phosphates in the soil due to overfertilization for periods of time, the reduction of fertilizer input due to the collapse the Eastern block and other factors contributed to this decrease. In developing countries, the demand for fertilizers is still increasing due to the growing population and emerging intensified agriculture which still needs to be established.
These imbalances in demand of fertilizers containing phosphates are also reported by Yang et al. 2019: As stated before the production of phosphate rock and the resulting fertilizer production is concentrated to a few countries, the demand though is present in every country practicing agriculture. This leads to dependencies on countries producing phosphate rock: Especially dependent on imports of fertilizers containing phosphates are sub-Saharan Africa (except South Africa), South American countries (except Venezuela and Peru), Middle- and Eastern European countries and South East Asia and Oceania.
Recycling phosphorus is a solution for industrialized countries to decrease the dependence on phosphate rock producing countries. In some cases the demand however cannot even be met by a 100% recycling efficiency: In some sub-Saharan developing countries phosphorus inputs need to increase by a factor of 2.3 to 11.7 to enable sufficient agricultural production to support the respective populations (Yang et al. 2019).
Future perspectives
As phosphate rock is a non-substitutable commodity, it is necessary to account for future perspectives on supply and demand. Easily accessible ore deposits are typically mined first (Cooper and Giurco 2009) and the average concentration and availability of phosphate rock has declined since the beginning of phosphate rock mining (van Kauwenbergh 2010). With a growing global population and rising standards of living, the demand of phosphate rock respectively fertilizers is ever increasing. According to Childers et al. 2011 global food production may need to increase by 70% to 100% with current projected population increases.
Supply
The future supply of phosphate rock is tightly connected with current reserves and resources, production volumes and recycling technologies. Table 1 shows the currently largest known reserves: As can be seen, Morocco and Western Sahara have the biggest reserves by a large margin.
Table 1: Largest phosphate rock reserves in thousand metric tons in 2021 (US Geological Survey 2022)
Country | Reserves |
United States | 1 000 000 |
Algeria | 2 200 000 |
Australia | 1 100 000 |
Brazil | 1 600 000 |
China | 3 200 000 |
Egypt | 2 800 000 |
Finland | 1 000 000 |
Jordan | 1 000 000 |
Morocco and Western Sahara | 50 000 000 |
Saudi Arabia | 1 400 000 |
South Africa | 1 600 000 |
There is no imminent physical scarcity in the near-and midterm future (Mew M. 2009; van Vuuren et al. 2010; van Kauwenbergh 2010). However, the formation of phosphate rock deposits by magmatic activity and sedimentary marine sequences took millions of years to form with formation rates being a lot slower than mining rates (Pufahl and Groat 2017). A prevalent term in the scientific community is peak phosphorus which is similarly to peak oil: Peak phosphorus refers to a year in the past/future when the phosphorus production has reached/reaches its peak. There is no consensus on the exact occurrence of a peak phosphorus globally in the future. According to Childers et al. 2011 it is bound to occur somewhere around the 2030s. This prediction however was made before newly detected reserves in Morocco and Western Sahara enlarged their reserves to around 50 million thousand metric tons of phosphate rock. Additionally, large phosphate deposits have been discovered recently on the continental shelf and on seamounts in the Atlantic- and Pacific Ocean (US Geological Survey 2022). Cordell and White 2011 summarize estimates from different authors regarding the year of depletion of phosphate rock reserves: Predictions are in between the year 2050 and 2410, so there is a great variety in estimations of phosphate rock reserves. However, at some point in the future phosphate rock resources will be depleted when current mining practices will be continued.
Recycling is therefore considered to be one of the best options to manage future phosphorus supply (Golroudbary et al. 2019). As before mentioned, there are many organic waste streams containing phosphates which could be recovered. One promising source is urban wastewater which potentially bears 1.2 g of phosphorus per person per day (Elser and Bennett 2011): Phosphates can be extracted from wastewater directly, they can be extracted from burned sludge ash or from dewatered sludge. Currently, the unfavorable economics of phosphorus recovery from wastewater hinder large scale applications (Mayer et al. 2016). Du et al. 2019 report the viability of steelmaking slag as a future source of phosphorus due to its quantity and quality.
However, to supply the necessary quantities of phosphorus from recycled sources Golroudbary et al. 2019 investigated the energy consumption therefore. They conclude that the energy demand might even be higher when phosphorus is mainly recovered from secondary sources in contrast to primary sources such as mining.
Demand
The future demand of phosphate rock is closely related to the growing global population. More food needs to be provided and the increasing standards of living in emerging countries require even more fertilizer because of changes in diets towards western standards. Accompanied by a growing global population which is estimated to be more than 10 billion people by the year of 2100 (United Nations 2022) a sustainable management of primary and secondary phosphorus reserves gains in importance. The necessity of phosphorus for living organisms and its non-substitutability will ensure an everlasting demand for this commodity.
Conclusion
The sustainable management of phosphate rock reserves and phosphates from secondary sources which are currently still accessible within/from the technosphere is inherently important for the future food security of humankind in the next few decades and centuries. The criticality assessment and perspective on the future availability and demand of phosphorus is therefore an essential management tool to ensure a sustainable society.
Bibliography
Al-Atrush, S. (2022): Saudi Arabia targets phosphates growth. Oil-rich kingdom looks to mining sector as it steps up diversification plans. Riyadh. Available online at https://www.ft.com/content/5d4654f4-9db5-41a8-b595-dd397a51d29e.
Belboom, S.; Szöcs, C.; Léonard, A. (2015): Environmental impacts of phosphoric acid production using di-hemihydrate process: a Belgian case study. In J. Clean. Prod. 108, 978–986.
Blengini, Gian Andrea; Nuss, Philip; Dewulf, Jo; Nita, Viorel; Peirò, Laura Talens; Vidal-Legaz, Beatriz et al. (2017): EU methodology for critical raw materials assessment: Policy needs and proposed solutions for incremental improvements. In Resources Policy 53, pp. 12–19. DOI: 10.1016/j.resourpol.2017.05.008.
Childers, Daniel L.; Corman, Jessica; Edwards, Mark; Elser, James J. (2011): Sustainability Challenges of Phosphorus and Food: Solutions from Closing the Human Phosphorus Cycle. In BioScience 61 (2), pp. 117–124. DOI: 10.1525/bio.2011.61.2.6.
Cooper, C.; Giurco, D. (2009): The mineral resources landscape – An expanded conceptualisation of minerals Sustainability.
Cooper, James; Lombardi, Rachel; Boardman, David; Carliell-Marquet, Cynthia (2011): The future distribution and production of global phosphate rock reserves. In Resources, Conservation and Recycling 57, pp. 78–86. DOI: 10.1016/j.resconrec.2011.09.009.
Cordell, D.; Rosemarin, A.; Schröder, J. J.; Smit, A. L. (2011): Towards global phosphorus security: a systems framework for phosphorus recovery and reuse options. In Chemosphere 84 (6), pp. 747–758. DOI: 10.1016/j.chemosphere.2011.02.032.
Cordell, D.; White, S. (2013): Sustainable phosphorus measures: Strategies and technologies for achieving phosphorus security. In Agronomy 3, pp. 86–116. Available online at http://www.mdpi.com/journal/agronomy.
Cordell, Dana; Drangert, Jan-Olof; White, Stuart (2009): The story of phosphorus: Global food security and food for thought. In Global Environmental Change 19 (2), pp. 292–305. DOI: 10.1016/j.gloenvcha.2008.10.009.
Cordell, Dana; White, Stuart (2011): Peak Phosphorus: Clarifying the Key Issues of a Vigorous Debate about Long-Term Phosphorus Security. In Sustainability 3 (10), pp. 2027–2049. DOI: 10.3390/su3102027.
Du, Chuan-Ming; Gao, Xu; Ueda, Shigeru; Kitamura, Shin-Ya (2019): Separation and recovery of phosphorus from steelmaking slag via a selective leaching–chemical precipitation process. In Hydrometallurgy 189, p. 105109. DOI: 10.1016/j.hydromet.2019.105109.
Edgerton, M. D. (2009): Increasing crop productivity to meet global needs for feed, food, and fuel plant. In Plant physiology 149 (1), pp. 7–13.
Elser, J.; Bennett, E. (2011): A broken biogeochemical cycle. In Nature 478, pp. 29–31. DOI: 10.1038/478029a.
Elser J.J.; Bracken, M.E.S.; Cleland, E. E.; Gruner, D. S.; Harpole, W. S.; Hillebrand, H. (2007): Global analysis of nitrogen and phosphorus limitation of primary producers in freshwater, marine, and terrestrial ecosystems. In Ecology Letters 10, pp. 1135–1142.
European Commission (2014): Report on Critical Raw Materials for the EU, Report of the Ad-hoc Working Group on Defining Critical Raw Materials.
European Commission (2015): Pursuant to Article 16 of Regulation (EC) No 648/2004 of the European Parliament and of the Council of 31 March 2004 on detergents, concerning the use of phosphorus in consumer automatic dishwasher detergents.
European Commission (2020): Study on the EU’s list of Critical Raw Materials – Final Report.
FAO (2006): Plant Nutrition for Food Security: A Guide for Integrated Nutrient Management. In FAO Fertilizer and Plant Nutrient Bulletin 16. FAO.
Filippelli, G. M. (2008): The Global Phosphorus Cycle: Past, Present, and Future. In Elements 4 (2), pp. 89–95. DOI: 10.2113/GSELEMENTS.4.2.89.
Golroudbary, Saeed Rahimpour; El Wali, Mohammad; Kraslawski, Andrzej (2019): Environmental sustainability of phosphorus recycling from wastewater, manure and solid wastes. In The Science of the total environment 672, pp. 515–524. DOI: 10.1016/j.scitotenv.2019.03.439.
Haase, Martina; Rösch, Christine; Ulrici, Olivier (2017): Feasibility study on the processing of surplus livestock manure into an organic fertilizer by thermal concentration – The case study of Les Plenesses in Wallonia. In Journal of Cleaner Production 161, pp. 896–907. DOI: 10.1016/j.jclepro.2017.05.207.
Holleman, A. F.; Wiberg, E.; Wiberg, N. (1985): Lehrbuch der Anorganischen Chemie. 91-100. Berlin: Walter de Gruyter.
Lamastra, L.; Suciu, Nicoleta Alina; Trevisan, Marco (2018): Sewage sludge for sustainable agriculture: contaminants’ contents and potential use as fertilizer. In Chem. Biol. Technol. Agric. 5 (10). DOI: 10.1186/s40538-018-0122-3.
Lauriente, D. H. (2003): Phosphate Rock. Stanford Research Institute.
Mayer, Brooke K.; Baker, Lawrence A.; Boyer, Treavor H.; Drechsel, Pay; Gifford, Mac; Hanjra, Munir A. et al. (2016): Total Value of Phosphorus Recovery. In Environmental science & technology 50 (13), pp. 6606–6620. DOI: 10.1021/acs.est.6b01239.
Metson, Geneviève S.; Bennett, Elena M.; Elser, James J. (2012): The role of diet in phosphorus demand. In Environ. Res. Lett. 7 (4), p. 44043. DOI: 10.1088/1748-9326/7/4/044043.
Mew, Michael C.; Steiner, Gerald; Geissler, Bernhard (2018): Phosphorus Supply Chain—Scientific, Technical, and Economic Foundations: A Transdisciplinary Orientation. In Sustainability 10 (1087). DOI: 10.3390/su10041087.
Mew M. (2009): Future phosphate rock production – peak or plateau? Available online at https://www.donau-uni.ac.at/dam/jcr:c6d9a9cd-d00e-4329-bdb0-b5c9115e03e2/Mew_Phosphate%20Rock,%20Peak%20or%20Plateau.pdf.
Michael, I.; Rizzo, L.; McArdell, C. S.; Manaia, C. M.; Merlin, C.; Schwartz, T. et al. (2013): Urban wastewater treatment plants as hotspots for the release of antibiotics in the environment: a review. In Water research 47 (3), pp. 957–995. DOI: 10.1016/j.watres.2012.11.027.
Nigg, Benno M.; Herzog, Walter (Eds.) (2007): Biomechanics of the musculo-skeletal system. Third edition.
Pearce, BinBin J.; Chertow, Marian (2017): Scenarios for achieving absolute reductions in phosphorus consumption in Singapore. In Journal of Cleaner Production 140, pp. 1587–1601. DOI: 10.1016/j.jclepro.2016.09.199.
Pufahl, Peir K.; Groat, Lee A. (2017): Sedimentary and Igneous Phosphate Deposits: Formation and Exploration: An Invited Paper. In Economic Geology 112 (3), pp. 483–516. DOI: 10.2113/econgeo.112.3.483.
Scholz, Roland W.; Ulrich, Andrea E.; Eilittä, Marjatta; Roy, Amit (2013): Sustainable use of phosphorus: a finite resource. In The Science of the total environment 461-462, pp. 799–803. DOI: 10.1016/j.scitotenv.2013.05.043.
Shiu, Huan-Yu; Lee, Mengshan; Chiueh, Pei-Te (2017): Water reclamation and sludge recycling scenarios for sustainable resource management in a wastewater treatment plant in Kinmen islands, Taiwan. In Journal of Cleaner Production 152, pp. 369–378. DOI: 10.1016/j.jclepro.2017.03.110.
Smil V. (2000): Phosphorus in the environment: Natural flows and human interferences. In Annual Review of Energy and the Environment 25, 53–88.
Stewart, William M.; Hammond, Lawrence L.; van Kauwenbergh, Steven J. (2005): Phosphorus: Agriculture and the Environment. Agronomy Mongraphs no. 46. American Society of Agronomy. Phosphorus as a Natural Resource.
United Nations (2022): World Population Prospects 2022. Department of Economic and Social Affairs, Population Division, Online Edition. Available online at https://population.un.org/wpp/Download/Standard/MostUsed/.
United Nations Statistics Division (2022): UN COMTRADE. International Merchandise Trade Statistics. Available online at https://comtrade.un.org/, checked on January 2023.
US Geological Survey (1994 – 2022): Phosphate Rock Statistics and Information.
US Geological Survey (2022): Phosphate Rock Statistics and Information. Available online at https://www.usgs.gov/centers/national-minerals-information-center/phosphate-rock-statistics-and-information, checked on January 2023.
van Enk, R. J.; Acera, L. K.; Schuiling, R. D.; Ehlert, P.; Wilt, J. G. de; van Haren, R.J.F. (2011): The Phosphate Balance: Current Developments and Future Outlook. InnovationNetwerk: Utrecht, The Netherlands.
van Kauwenbergh, S. (2010): World phosphate rock reserves and resources, 2010.
van Vuuren, D. P.; Bouwman, A. F.; Beusen, A.H.W. (2010): Phosphorus demand for the 1970–2100 period: a scenario analysis of resource depletion. In Glob Environ Chang 20 (3), pp. 428–439.
Walan, P. (2013): Modeling of Peak Phosphorus. A Study of Bottlenecks and Implications for Future Production. Uppsala Universitet.
Yang, Haozhe; Liu, Ying; Liu, Junfeng; Meng, Jing; Hu, Xiurong; Tao, Shu (2019): Improving the Imbalanced Global Supply Chain of Phosphorus Fertilizers. In Earth’s Future 7 (6), pp. 638–651. DOI: 10.1029/2018EF001005.
Ye, Y.; Ngo, H. H.; Guo, W.; Liu, Y.; Li, J.; Zhang, X.; Jia, H. (2017): Insight into chemical phosphate recovery from municipal wastewater. In Sci. Total Environ. 576, pp. 159–171.